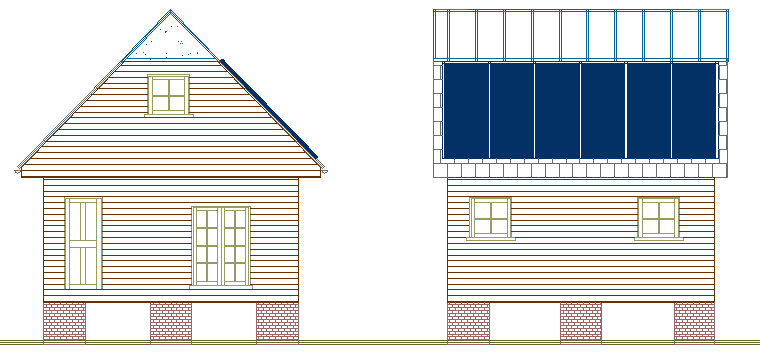
A
nicely integrated solar home - as far as we know the 1st proposed
IS
FREE CENTRAL HEATING POSSIBLE?
The
answer to that is not simple and it all depends on the design of any
system. in our opinion, a system needs to be fully integrated to stand a
chance of providing free heating - that is to say affordable heating for
a sustainable future. Nothing is totally free because of the set up and
long term maintenance costs. Thus, cost-effectiveness is more to the
point, and cost effectiveness depends on the mix of technology. All
design in of course a compromise. The typical systems described below
are not integrated as per the Utopia Tristar™ system - but they show
the current state of play - and that is not bad where free hot water is
the result, it is just not sufficient to heat a home.
SOLAR
WATER HEATING (SWH)
SHW systems
are now a well developed renewable energy technology that has been well established for many years. SWH has been widely used in Australia, Austria, China, Cyprus, Greece, India, Israel, Japan and
Turkey because of the high local sunshine. There are two main types:
A - In a "close-coupled" SWH system the storage tank is horizontally mounted immediately above the solar collectors on the roof. No pumping is required as the hot water naturally rises into the tank through thermosiphon flow.
Such a system is not suitable for economical volume storage, due to the
weight of the storage medium and the increased structural costs that
would entail.
B
- In a "pump-circulated" system the storage tank is ground- or floor-mounted and is below the level of the collectors; a circulating pump moves water or heat transfer fluid between the tank and the collectors.
This system does not stress a building structure, but, would involve
considerable ground works if conventional tanks were employed - so would
be expensive.
SWH systems are designed to deliver hot water for most of the year. However, in winter there sometimes may not be sufficient solar heat gain to deliver sufficient hot water. In this case a gas or electric booster is normally used to heat the water.
Thus, we say 'virtually' free heating - because of the unpredictability
of winter.
OVERVIEW
We
will concentrate on water systems here, because air and subsurface stone
heat stores are once again impractical in cost terms. Hot water heated by the sun is used in many ways.
The best known is for residential domestic hot water for washing and
baths. Designs suitable for hot climates can be much simpler and cheaper, and
are considered an appropriate technology for these places, but we want
to extend the technology for the UK. The global solar thermal market is dominated by China, Europe, Japan and India.
BASIC
WORKINGS - TYPICAL SYSTEMS
In order to heat water using solar energy, a collector, often fastened to a roof or a wall facing the sun, heats working fluid that is either pumped (active system) or driven by natural convection (passive system) through it. The collector could be made of a simple glass-topped insulated box with a flat solar absorber made of sheet metal, attached to copper heat exchanger pipes and dark-colored, or a set of metal tubes surrounded by an evacuated (near vacuum) glass cylinder. In industrial cases a parabolic mirror can concentrate sunlight on the tube. Heat is stored in a hot water storage tank. The volume of this tank needs to be larger with solar heating systems in order to allow for bad
weather, and because the optimum final temperature for the solar
collector is lower than a typical immersion or combustion heater.
The heat transfer fluid (HTF) for the absorber may be the hot water from the tank, but more commonly (at least in active systems) is a separate loop of fluid containing anti-freeze and a corrosion inhibitor which delivers heat to the tank through a heat exchanger (commonly a coil of copper heat exchanger tubing within the tank). Copper is an important component in solar thermal heating and cooling systems because of its high heat conductivity, resistance to atmospheric and water corrosion, sealing and joining by soldering, and mechanical strength. Copper is used both in receivers and primary circuits (pipes and heat exchangers for water tanks).
Another lower-maintenance concept is the 'drain-back': no anti-freeze is required; instead, all the piping is sloped to cause water to drain back to the tank. The tank is not pressurized and is open to atmospheric pressure. As soon as the pump shuts off, flow reverses and the pipes are empty before freezing could occur.
Residential solar thermal installations fall into two groups: passive (sometimes called "compact") and active (sometimes called "pumped") systems. Both typically include an auxiliary energy source (electric heating element or connection to a gas or fuel oil central heating system) which is activated when the water in the tank falls below a minimum temperature setting such as 55 °C. Hence, hot water is always available. The combination of solar water heating and using the back-up heat from a wood stove chimney to heat water can enable a hot water system to work all year round in cooler climates, without the supplemental heat requirement of a solar water heating system being met with fossil fuels or
electricity.
PERCENTAGE
EFFECTIVENESS
When a solar water heating and hot-water central heating system are used in conjunction, solar heat will either be concentrated in a pre-heating tank that feeds into the tank heated by the central heating, or the solar heat exchanger will replace the lower heating element and the upper element will remain in place to provide for any heating that solar cannot provide. However, the primary need for central heating is at night and in winter when solar gain is lower. Therefore, solar water heating for washing and bathing is often a better application than central heating because supply and demand are better matched. In many climates, a solar hot water system can provide up to 85% of domestic hot water energy. This can include domestic non-electric concentrating solar thermal systems. In many northern European countries, combined hot water and space heating systems (solar
combi-systems) are used to provide 15 to 25% of home heating energy.
The best examples of solar heating in use are in Israel and Cyprus, being the per capita leaders in the use of solar water heating systems with over 30%–40% of homes using them.
Imagine the energy savings for those countries.
Flat plate solar systems were perfected and used on a very large scale in Israel. In the 1950s there was a fuel shortage in the new Israeli state, and the government forbade heating water between 10 pm and 6 am. Levi Yissar built the first prototype Israeli solar water heater and in 1953 he launched the NerYah Company, Israel's first commercial manufacturer of solar water heating. Despite the abundance of sunlight in Israel, solar water heaters were used by only 20% of the population by 1967. Following the energy crisis in the 1970s, in 1980 the Israeli Knesset passed a law requiring the installation of solar water heaters in all new homes (except high towers with insufficient roof area). As a result, Israel is now the world leader in the use of solar energy per capita with 85% of the households today using solar thermal systems (3% of the primary national energy consumption), estimated to save the country 2 million barrels (320,000 m3) of oil a year, the highest per capita use of solar energy in the world.
In 2005, Spain became the first country in the world to require the installation of photovoltaic electricity generation in new buildings, and the second (after Israel) to require the installation of solar water heating systems in 2006.
TYPES
OF SOLAR WATER HEATING SYSTEMS
Direct or open loop systems circulate potable water through the collectors. They are relatively cheap but can have the following drawbacks:
1. They offer little or no overheat protection unless they have a heat export pump.
2. They offer little or no freeze protection, unless the collectors are freeze-tolerant.
3. Collectors accumulate scale in hard water areas, unless an ion-exchange softener is used.
Until the advent of freeze-tolerant solar collectors, they were not considered suitable for cold climates since, in the event of the collector being damaged by a freeze, pressurized water lines will force water to gush from the freeze-damaged collector until the problem is noticed and rectified.
Indirect or closed loop systems use a heat exchanger that separates the potable water from the fluid, known as the "heat-transfer fluid" (HTF), that circulates through the collector. The two most common HTFs are water and an antifreeze/water mix that typically uses non-toxic propylene glycol. After being heated in the panels, the HTF travels to the heat exchanger, where its heat is transferred to the potable water. Though slightly more expensive, indirect systems offer freeze protection and typically offer overheat protection as well.
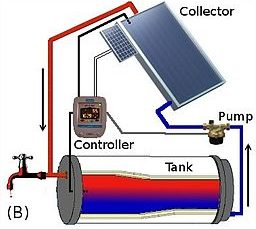
PASSIVE & ACTIVE SYSTEMS
Passive systems rely on heat-driven convection or heat pipes to circulate water or heating fluid in the system. Passive solar water heating systems cost less and have extremely low or no maintenance, but the efficiency of a passive system is significantly lower than that of an active system, and overheating and freezing are major concerns.
Active systems use one or more pumps to circulate water and/or heating fluid in the system.
Though slightly more expensive, active systems offer several advantages:
* The storage tank can be situated lower than the collectors, allowing increased freedom in system design and allowing pre-existing storage tanks to be used.
* The storage tank can always be hidden from view.
* The storage tank can be placed in conditioned or semi-conditioned space, reducing heat loss.
* Drainback tanks can be used.
* Superior efficiency.
* Increased control over the system.
Modern active solar water systems have electronic controllers that offer a wide range of functionality, such as the modification of settings that control the system, interaction with a backup electric or gas-driven water heater, calculation and logging of the energy saved by a SWH system, safety functions, remote access, and various informative displays, such as temperature readings.
The most popular pump controller is a differential controller that senses temperature differences between water leaving the solar collector and the water in the storage tank near the heat exchanger. In a typical active system, the controller turns the pump on when the water in the collector is about 8–10 °C warmer than the water in the tank, and it turns the pump off when the temperature difference approaches 3–5 °C. This ensures the water always gains heat from the collector when the pump operates and prevents the pump from cycling on and off too often. (In direct systems this "on differential" can be reduced to around 4C because there is no heat exchanger impediment.)
Some active SWH systems use energy obtained by a small photovoltaic (PV) panel to power one or more variable-speed DC pump(s). In order to ensure proper performance and longevity of the pump(s), the DC-pump and PV panel must be suitably matched. Some PV pumped solar thermal systems are of the antifreeze variety and some use freeze-tolerant solar collectors. The solar collectors will almost always be hot when the pump(s) are operating (i.e. when the sun is bright), and some do not use solar controllers. Sometimes, however, a differential controller (that can also be powered by the DC output of a PV panel) is used to prevent the operation of the pumps when there is sunlight to power the pump but the collectors are still cooler than the water in storage. One advantage of a PV-driven system is that solar hot water can still be collected during a power outage if the Sun is shining. Another advantage is that the operational carbon clawback of using mains pumped solar thermal (which typically negates up to 23% of its carbon savings) is completely avoided.
An active solar water heating system can also be equipped with a bubble pump (also known as geyser pump) instead of an electric pump. A bubble pump circulates the heat transfer fluid (HTF) between collector and storage tank using solar power and without any external energy source and is suitable for flat panel as well as vacuum tube systems. In a bubble pump system, the closed HTF circuit is under reduced pressure, which causes the liquid to boil at low temperature as it is heated by the sun. The steam bubbles form a geyser pump, causing an upward flow. The system is designed such that the bubbles are separated from the hot fluid and condensed at the highest point in the circuit, after which the fluid flows downward towards the heat exchanger caused by the difference in fluid levels. The HTF typically arrives at the heat exchanger at 70 °C and returns to the circulating pump at 50 °C. In frost prone climates the HTF is water with propylene glycol anti-freeze added, usually in the ratio of 60 to 40. Pumping typically starts at about 50 °C and increases as the sun rises until equilibrium is reached, which depends on the efficiency of the heat exchanger, the temperature of the water being heated, and the total solar energy available.
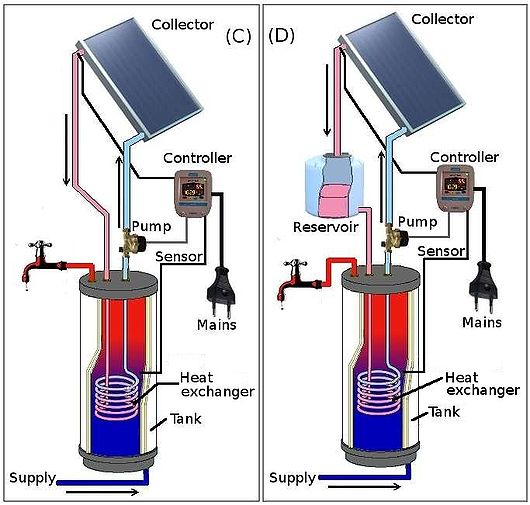
PASSIVE DIRECT SYSTEMS
An integrated collector storage (ICS or Batch Heater) system uses a tank that acts as both storage and solar collector. Batch heaters are basically thin rectilinear tanks with a glass side facing the position of the sun at noon. They are simple and less costly than plate and tube collectors, but they sometimes require extra bracing if installed on a roof (since they are heavy when filled with water [400–700 lbs],) suffer from significant heat loss at night since the side facing the sun is largely uninsulated, and are only suitable in moderate climates.
A convection heat storage unit (CHS) system is similar to an ICS system, except the storage tank and collector are physically separated and transfer between the two is driven by convection. CHS systems typically use standard flat-plate type or evacuated tube collectors, and the storage tank must be located above the collectors for convection to work properly. The main benefit of a CHS systems over an ICS system is that heat loss is largely avoided since (1) the storage tank can be better insulated, and (2) since the panels are located below the storage tank, heat loss in the panels will not cause convection, as the cold water will prefer to stay at the lowest part of the system.
TYPES OF COLLECTOR USED FOR SWH SYSTEMS
Solar thermal collectors capture and retain heat from the sun and use it to heat a liquid. Two important physical principles govern the technology of solar thermal collectors:
Any hot object ultimately returns to thermal equilibrium with its environment, due to heat loss from the hot object. The processes that result in this heat loss are conduction, convection and
radiation. The efficiency of a solar thermal collector is directly related to heat losses from the collector surface (efficiency being defined as the proportion of heat energy that can be retained for a predefined period of time). Within the context of a solar collector, convection and radiation are the most important sources of heat loss. Thermal insulation is used to slow down heat loss from a hot object to its environment. This is actually a direct manifestation of the Second law of thermodynamics but we may term this the 'equilibrium effect'.
Heat is lost more rapidly if the temperature difference between a hot object and its environment is larger. Heat loss is predominantly governed by the thermal gradient between the temperature of the collector surface and the ambient temperature. Conduction, convection, and radiation all occur more rapidly over large thermal gradients. We may term this the 'delta-t effect'.
The most simple approach to solar heating of water is to simply mount a metal tank filled with water in a sunny place. The heat from the sun would then heat the metal tank and the water inside. Indeed, this was how the very first SWH systems worked more than a century
ago. However, this setup would be inefficient due to an oversight of the equilibrium effect, above: as soon as heating of the tank and water begins, the heat gained starts to be lost back into the environment, and this continues until the water in the tank reaches the ambient temperature. The challenge is therefore to limit the heat loss from the tank, thus delaying the time when thermal equilibrium is regained.
ICS or batch collectors reduce heat loss by placing the water tank in a thermally insulated box. This is achieved by encasing the water tank in a glass-topped box that allows heat from the sun to reach the water tank. However, the other walls of the box are thermally insulated, reducing convection as well as radiation to the environment. In addition, the box can also have a reflective surface on the inside. This reflects heat lost from the tank back towards the tank. In a simple way one could consider an ICS solar water heater as a water tank that has been enclosed in a type of 'oven' that retains heat from the sun as well as heat of the water in the tank. Using a box does not eliminate heat loss from the tank to the environment, but it largely reduces this loss.
Standard ICS collectors have a characteristic that strongly limits the efficiency of the collector: a small surface-to-volume
ratio. Since the amount of heat that a tank can absorb from the sun is largely dependent on the surface of the tank directly exposed to the sun, it follows that a small surface would limit the degree to which the water can be heated by the sun. Cylindrical objects such as the tank in an ICS collector inherently have a small surface-to-volume ratio and most modern collectors attempt to increase this ratio for efficient warming of the water in the tank. There are many variations on this basic design, with some ICS collectors comprising several smaller water containers and even including evacuated glass tube technology, a type of ICS system known as an Evacuated Tube Batch (ETB) collector.
Flat plate collectors are an extension of the basic idea to place a collector in an 'oven'-like box with glass in the direction of the
Sun. Most flat plate collectors have two horizontal pipes at the top and bottom, called headers, and many smaller vertical pipes connecting them, called risers. The risers are welded (or similarly connected) to thin absorber fins. Heat-transfer fluid (water or water/antifreeze mix) is pumped from the hot water storage tank (direct system) or heat exchanger (indirect system) into the collectors' bottom header, and it travels up the risers, collecting heat from the absorber fins, and then exits the collector out of the top header. Serpentine flat plate collectors differ slightly from this "harp" design, and instead use a single pipe that travels up and down the collector. However, since they cannot be properly drained of water, serpentine flat plate collectors cannot be used in drainback systems.
The type of glass used in flat plate collectors is almost always low-iron, tempered glass. Being tempered, the glass can withstand significant hail without breaking, which is one of the reasons that flat-plate collectors are considered the most durable collector type.
Unglazed or formed collectors are similar to flat-plate collectors, except they are not thermally insulated nor physically protected by a glass panel. Consequently these types of collectors are much less efficient for domestic water heating. For pool heating applications, however, the water being heated is often colder than the ambient roof temperature, at which point the lack of thermal insulation allows additional heat to be drawn from the surrounding environment.
Evacuated tube collectors (ETC) are a way in which heat loss to the environment, inherent in flat plates, has been reduced. Since heat loss due to convection cannot cross a vacuum, it forms an efficient isolation mechanism to keep heat inside the collector pipes. Since two flat sheets of glass are normally not strong enough to withstand a vacuum, the vacuum is rather created between two concentric tubes. Typically, the water piping in an ETC is therefore surrounded by two concentric tubes of glass with a vacuum in between that admits heat from the sun (to heat the pipe) but which limits heat loss back to the environment. The inner tube is coated with a thermal absorbent. Life of the vacuum varies from collector to collector, anywhere from 5 years to 15 years.
Flat plate collectors are generally more efficient than ETC in full sunshine conditions. However, the energy output of flat plate collectors is reduced slightly more than evacuated tube collectors in cloudy or extremely cold conditions. Most ETCs are made out of annealed glass, which is susceptible to hail, breaking in roughly golf ball -sized hail. ETCs made from "coke glass," which has a green tint, are stronger and less likely to lose their vacuum, but efficiency is slightly reduced due to reduced transparency.
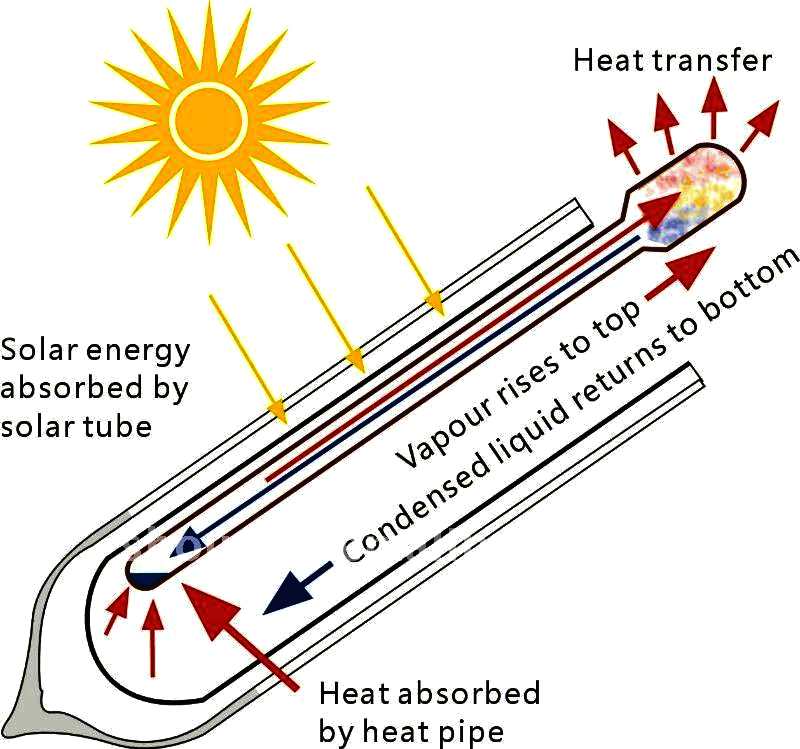
ECONOMICS - ENERGY COLLECTED v SYSTEM COSTS
ENERGY PRODUCTION
The amount of heat delivered by a solar water heating system depends primarily on the amount of heat delivered by the sun at a particular place (the insolation). In tropical places the insolation can be relatively high, e.g. 7 kW.h/m2 per day, whereas the insolation can be much lower in temperate areas where the days are shorter in winter, e.g. 3.2 kW.h/m2 per day. Even at the same latitude the average insolation can vary a great deal from location to location due to differences in local weather patterns and the amount of overcast. Useful calculators for estimating insolation at a site can be found with the Joint Research Laboratory of the European Commission and the American National Renewable Energy Laboratory.
Below is a table that gives a rough indication of the specifications and energy that could be expected from a solar water heating system involving some 2 m2 of absorber area of the collector, demonstrating two evacuated tube and three flat plate solar water heating systems. Certification information or figures calculated from those data are used. The bottom two rows give estimates for daily energy production (kW.h/day) for a tropical and a temperate scenario. These estimates are for heating water to 50 °C above ambient temperature.
With most solar water heating systems, the energy output scales linearly with the surface area of the absorbers. Therefore, when comparing figures, take into account the absorber area of the collector because collectors with less absorber area yield less heat, even within the 2 m2 range. Specifications for many complete solar water heating systems and separate solar collectors can be found at Internet site of the SRCC.
TABLE - Daily
energy production (kWth.h) of five solar thermal
systems.
Technology |
Flat
plate |
Flat
plate |
Flat
plate |
Evac
x20 tube |
Evac
x20 tube |
Configuration |
Direct active |
Thermosiphon |
Indirect active |
Indirect active |
Direct active |
Overall size (m2) |
2.49 |
1.98 |
1.87 |
2.85 |
2.97 |
Absorber size
(m2) |
2.21 |
1.98 |
1.72 |
2.85 |
2.96 |
Maximum
efficiency |
0.68 |
0.74 |
0.61 |
0.57 |
0.46 |
Energy
production (kW.h/day):
– Insolation 3.2 kW.h/m2/day (temperate)
– e.g. Zurich, Switzerland |
5.3 |
3.9 |
3.3 |
4.8 |
4.0 |
– Insolation
6.5 kW.h/m2/day (tropical)
– e.g. Phoenix, USA |
11.2 |
8.8 |
7.1 |
9.9 |
8.4 |
The figures are fairly similar between the above collectors, yielding some 4 kW.h/day in a temperate climate and some 8 kW.h/day in a more tropical climate when using a collector with an absorber area of about 2m2 in size. In the temperate scenario this is sufficient to heat 200 litres of water by some 17 °C. In the tropical scenario the equivalent heating would be by some 33 °C. Many thermosiphon systems are quite efficient and have comparable energy output to equivalent active systems. The efficiency of evacuated tube collectors is somewhat lower than for flat plate collectors because the absorbers are narrower than the tubes and the tubes have space between them, resulting in a significantly larger percentage of inactive overall collector area. Some methods of
comparison calculate the efficiency of evacuated tube collectors based on the actual absorber area and not on the 'roof area' of the system as has been done in the above table. The efficiency of the collectors becomes lower if one demands water with a very high temperature.
SYSTEM COSTS
In sunny, warm locations, where freeze protection is not necessary, an ICS (batch type) solar water heater can be extremely cost effective. In higher latitudes, there are often additional design requirements for cold weather, which add to system complexity. This has the effect of increasing the initial cost (but not the life-cycle cost) of a solar water heating system, to a level much higher than a comparable hot water heater of the conventional type. The biggest single consideration is therefore the large initial financial outlay of solar water heating systems. Offsetting this expense can take several years and the payback period is longer in temperate environments where the insolation is less intense.
When calculating the total cost to own and operate, a proper analysis will consider that solar energy is free, thus greatly reducing the operating costs, whereas other energy sources, such as gas and electricity, can be quite expensive over time. Thus, when the initial costs of a solar system are properly financed and compared with energy costs, then in many cases the total monthly cost of solar heat can be less than other more conventional types of hot water heaters (also in conjunction with an existing hot water heater). At higher latitudes, solar heaters may be less effective due to lower solar energy, possibly requiring larger and/or dual-heating systems. In addition, government incentives can be significant.
The calculation of long term cost and payback period for a household SWH system depends on a number of factors. Some of these are:
* Price of purchasing solar water heater (more complex systems are more expensive)
* Efficiency of SWH system purchased
* Installation cost
* Price of electricity use for mains pumping (if this is used)
* Price of water heating fuel (e.g. gas or electricity) saved per kW.h
* Amount of water heating fuel used per month by a household
* Upfront state or government subsidy for installation of a solar water heater
* Recurrent or annual tax rebates or subsidy for operating renewable energy
* Annual maintenance cost of SWH system (e.g. antifreeze or pump replacements)
* Savings in annual maintenance of conventional (electric/gas/oil) water heating system
The following table gives some idea of the cost and payback period to recover the costs. It does not take into account annual maintenance costs, annual tax rebates and installation costs. However, the table does give an indication of the total cost and the order of magnitude of the payback period. The table assumes an energy savings of 200 kW.h per month (about 6.57 kW.h/day) due to SWH. Unfortunately payback times can vary greatly due to regional sun, extra cost due to frost protection needs of collectors, household hot water use etc. so more information may be needed to get accurate estimates for individual households and regions. For instance in central and southern Florida the payback period could easily be 7 years or less rather than the 12.6 years indicated on the chart for the US
CHART - COST v PAYBACK RESIDENTILA SWH SYST with savings 200kW h/month
(using 2010 data)
Country |
Currency |
Syst
cost |
Subsidy(%) |
Effective
cost |
Electricity
cost/kW.h |
Electricity
savings/month |
Payback
in years |
Brazil |
BRL |
2500 |
0 |
2500 |
0.25 |
50 |
4.2 |
South
Africa |
ZAR |
14000 |
15 |
11900 |
0.9 |
180 |
5.5 |
Australia |
AUD |
5000 |
40 |
3000 |
0.18 |
36 |
6.9 |
Belgium |
EUR |
4000 |
50 |
2000 |
0.1 |
20 |
8.3 |
United
States |
USD |
5000 |
30 |
3500 |
0.1158 |
23.16 |
12.6 |
United
Kingdom |
GBP |
4800 |
0 |
4800 |
0.11 |
22 |
18.2 |
Two points are clear from the above table. Firstly, the payback period is shorter in countries with a large amount of insolation and even in parts of the same country with more insolation. This is evident from the payback period less than 10 years in most southern hemisphere countries, listed above. This is partly because of good sunshine, allowing users in those countries to need smaller systems than in temperate areas. Secondly, even in the northern hemisphere countries where payback periods are often longer than 10 years, solar water heating is financially extremely efficient. This is partly because the SWH technology is efficient in capturing irradiation. The payback period for photovoltaic systems is much longer. In many cases the payback period for a SWH system is shortened if it supplies all or nearly all of the warm water requirements used by a household. Many SWH systems supply only a fraction of warm water needs and are augmented by gas or electric heating on a daily basis, thus extending the payback period of such a system.
Solar leasing is now available in Spain for solar water heating systems from Pretasol with a typical system costing around 59 euros and rising to 99 euros per month for a system that would provide sufficient hot water for a typical family home of six persons. The payback period would be five years.
Australia has instituted a system of Renewable Energy Credits, based on national renewable energy targets. This expands an older system based only on rebates.
STANDARDS - EUROPE
EN 806: Specifications for installations inside buildings conveying water for human consumption. General.
EN 1717: Protection against pollution of potable water in water installations and general requerements of devices to prevent pollution by backflow.
EN 60335: Specification for safety of household and similar electrical appliances. (2–21)
UNE 94002:2005 Thermal solar systems for domestic hot water production. Calculation method for heat demand.
STANDARDS
- UNITED STATES
OG-300: OG-300 Certification of Solar Water Heating Systems.
DO IT YOURSELF (DIY) SYSTEMS
People have begun building their own (small-scale) solar water heating systems from scratch or buying kits. Plans for solar water heating systems are available on the Internet. and people have set about building them for their own domestic requirements. DIY SWH systems are usually cheaper than commercial ones, and they are used both in the developed and developing world.
System specification and installation:
* Except in rare instances it will be insufficient to install a SWH system with no electrical or gas or other fuel backup. Many SWH systems have a back-up electric heating element in the integrated tank, the operation of which may be necessary on cloudy days to ensure a reliable supply of hot water.
* The temperature stability of a system is dependent on the ratio of the volume of warm water used per day as a fraction of the size of the water reservoir/tank that stores the hot water. If a large proportion of hot water in the reservoir is used each day, a large fraction of the water in the reservoir needs to be heated. This brings about significant fluctuations in water temperature every day, with possible risks of overheating or underheating, depending on the design of the system. Since the amount of heating that needs to take place every day is proportional to hot water usage and not to the size of the reservoir, it is desirable to have a fairly large reservoir (i.e. equal to or greater than daily usage,) which will help prevent fluctuations in water temperature.
* If ample storage is pre-existing or can otherwise be reasonably acquired, a large SWH system is more efficient economically than a small system. This is because the price of a system is not linearly proportional to the size of the collector array, so the price per square meter of collector is cheaper in a larger system. If this is the case, it pays to use a system that covers nearly all of the domestic hot water needs, and not only a small fraction of the needs. This facilitates more rapid cost recovery.
* Not all installations require new replacement solar hot water stores. Existing stores may be large enough and in suitable condition. Direct systems can be retrofitted to existing stores while indirect systems can be also sometimes be retrofitted using internal and external heat exchangers.
* The installation of a SWH system needs to be complemented with efficient insulation of all the water pipes connecting the collector and the water storage tank, as well as the storage tank (or "geyser") and the most important warm water outlets. The installation of efficient lagging significantly reduces the heat loss from the hot water system. The installation of lagging on at least two meters of pipe on the cold water inlet of the storage tank reduces heat loss, as does the installation of a "geyser blanket" around the storage tank (if inside a roof). In cold climates the installation of lagging and insulation is often performed even in the absence of a SWH system.
* The most efficient PV pumps are designed to start very slowly in very low light levels, so if connected uncontrolled, they may cause a small amount of unwanted circulation early in the morning – for example when there is enough light to drive the pump but while the collector is still cold. To eliminate the risk of hot water in the storage tank from being cooled that way this is very important. solar controller may be required.
* The modularity of an evacuated tube collector array allows the adjustment of the collector size by removing some tubes or their heat pipes. Budgeting for a larger than required array of tubes therefore allows for the customisation of collector size to the needs of a particular application, especially in warmer climates.
* Particularly in locations further towards the poles than 45 degrees from the equator, roof mounted sun facing collectors tend to outperform wall mounted collectors in terms of total energy output. However, it is total useful energy output which usually matters most to consumers. So arrays of sunny wall mounted steep collectors can sometimes produce more useful energy because there can be a small increase in winter gain at the expense of a large unused summer surplus.
JOIN
IN
Join
us in the drive for a green economy. Email
or call to register your interest in these exciting sustainable
developments for a better Britain.
STATE
OF THE ART
You
should note from all the systems looked at on this page, that none is an
integrated solution. They are all add-ons, or aftermarket accessories.
To be truly cost effective, such a system must form part of the design
of the house
DOMESTIC
RHI SCHEME
The UK Government published its consultation on domestic RHI in September 2012. The key proposals in the consultation are:
Indicative tariff ranges for air source heat pumps (6.9-11.5p/kWh), biomass boilers (5.2-8.7p/kWh), ground source heat pumps (12.5-17.3p/kWh) and solar thermal technologies (17.3p/kWh) that are MCS certified and meet relevant required standards, including specific emission limits for biomass systems.
Payments for householders over seven years for each kWh of heat produced for the expected lifetime of the renewable technology and based on deemed heat usage.
Tariff levels set to provide a better return for householders living off the gas grid.
Minimum energy efficiency requirements based on Green Deal assessments.
The UK government has also confirmed that people who have installed equipment under RHPP 1 or 2 will be eligible for support through the RHI providing they meet the eligibility criteria of the full RHI scheme.
http://www.energysavingtrust.org.uk/Generating-energy/Getting-money-back/Renewable-Heat-Incentive-RHI
|